
The X-ray tube is the heart of an X-ray diffractometer. Inside, a tungsten filament (cathode) is heated so some electrons have enough energy to leave its surface. The power supply puts a high voltage between the filament (high negative potential) and the anode metal (ground potential). Electrons from the filament are accelerated by the electric potential to the anode, striking it with an energy equal to the tube voltage. The shaped electron beam hits the target along a line about 0.4 by 12 mm.

The tube fits into a housing above the high voltage generator box. In our system, one line focus window is left uncovered, so X-rays can shine onto your sample. Copper X-rays are produced in three wavelengths: Cu Kα1 and Cu Kα2 have similar wavelengths and will not be discussed separately here, and Cu Kβ, which has a somewhat shorter wavelength and so higher energy. Having Kβ and Kα X-rays together doubles the number of diffraction peaks, complicating the pattern but adding no additional information. In our instrument the Kβ X-rays are removed with a nickel foil filter, which strongly absorbs Cu Kβ X-rays but is nearly transparent to Kα X-rays. The following four figures show how it works.
When energetic electrons strike the anode, they knock electrons from the inner electron shells of the metal atoms. Electrons in the outer shells then “fall down” to fill the vacant inner shell, losing energy by emitting X-ray photons of characteristic energy and wavelength. The X-rays radiate in all directions, but some pass through four thin beryllium windows. Because the electron beam strikes the target along a line, if you could look through either of the two beryllium windows that are parallel to the line focus you would see X-rays shining from a thin line. These are “line focus” windows, used for goniometer-type powder diffractometry. If you could look through the other two windows that are perpendicular to the line focus, you would see X-rays emitted from what appears to be a point, which is the foreshortened perspective view of the line. These are “point focus” windows, used for powder and single crystal cameras.

As electrons hit the copper anode, they knock K-shell electrons from copper atoms. Other electrons lose energy to fill the K-shell vacancies, emitting X-rays. These Kβ and Kα (combined Kα1 and Kα2) X-rays have characteristic wavelengths of 1.393 Å and 1.542 Å; (energies shown in the figure). With no filter the Kβ X-rays reach your sample. The continuum X-rays have several sources. They are unwanted but have to be put up with on our instrument.

The Cu K excitation energy is 8.979 kV, corresponding to a wavelength of 1.381Å;. Because the Cu K excitation energy is higher than the energy of either Cu X-ray lines, Cu radiation is only weakly absorbed by a copper filter. As a result, a copper filter slightly reduces the height of both peaks.
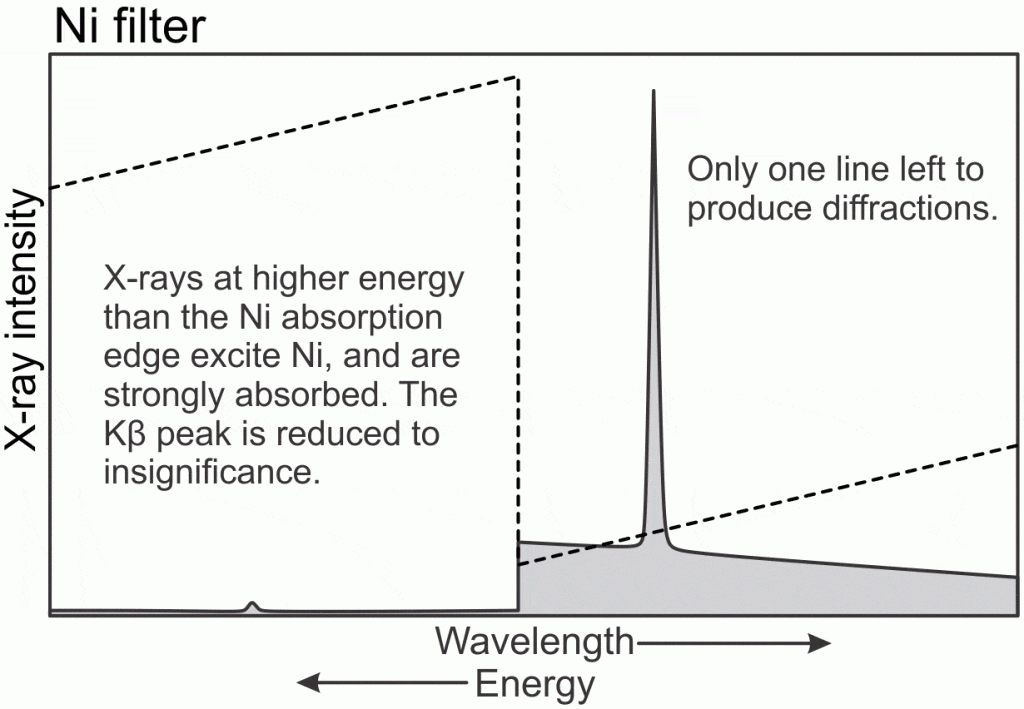
Ni has a K excitation energy at 8.333 kV, or 1.488 Å;. This energy is between the Cu Kα and Kβ peaks, so the higher energy Kβ X-rays excite the Ni filter and are strongly absorbed. The Cu Kα X-rays are not energetic enough to excite Ni, so they are only weakly absorbed. The result is a modest reduction in Cu Kα X-ray intensity, and an almost complete elimination of the Kβ X-rays. Elimination of Kβ X-rays results in a much simpler diffraction pattern, so a Ni filter is usually used with Cu X-ray tubes in systems like ours.

The Co K excitation energy at 7.708 kV corresponds to 1.608 Å, is lower in energy than either of the Cu X-ray lines. Both Cu lines therefore excite Co K-shell electrons, so both are strongly absorbed. The result is that both Cu X-ray peaks are greatly reduced in size after passing through Co foil, resulting in a diffraction pattern with no peaks at all.